Energy Harvesting: Perpetual Power, Maintenance Free
For design engineers, success with micro-energy harvesting requires equal parts knowledge and willingness to experiment.
Latest News
March 1, 2011
By Barbara G. Goode
![]() Powercast’s Lifetime Power Energy Harvesting Development Kit for Wireless Sensors, created with development support from Microchip, enables wireless, RF-based battery-free operation of sensor nodes (each detects temperature, humidity and light, and an additional parameter via an external port) at a distance of 40 to 45 ft. |
Energy harvesting is a still-new concept—embodied by a range of technologies—that lets design engineers create versatile, autonomous systems and products that operate free from power maintenance. A well-known example is Seiko’s Kinetic line of watches, which are powered by the movement of the wearer’s body. Less well known, but increasing in number, are installations such as the one devised by Leviton Manufacturing, a supplier of lighting energy management systems. Leviton’s engineering team has paired miniature solar cells with low-power consumption devices and designs to produce an effective, self-powered wireless occupancy sensor and lighting control system able to “keep the lights on when there is occupancy and allow the lights to go off after 2 to 30 minutes with no occupancy,” according to Dan Wright, lead electrical engineer, and Paul Maddox, director of engineering.
“There’s certainly a growing interest in energy harvesting, and it’s becoming widespread over a number of industries,” says Harry Ostaffe, vice president, marketing and business development for Powercast Corp., maker of products that convert radio frequency (RF) energy into DC power. Also called power scavenging, the approach involves collecting ambient energy that is normally overlooked, untapped and wasted—for instance, vibration energy from machines and rail cars, thermal energy from industrial processes, or radiant energy from lighting. The harvesters convert the power for use by devices able to operate on miserly amounts of power. Such devices include radio frequency identification (RFID) tags, which is used for location tracking, security, etc. Others examples include switches, sensors (especially wireless sensors, designed to send their data by way of radio waves), low-power displays and more.
“Energy harvesting is generally divided into two camps,” explains Keith J. Abate Sr., director of business development for Perpetuum, which designs and manufactures vibration energy harvesters: Macro systems generate > 1ma of power output (think solar arrays and windmills) and micro systems generate < 1ma. Generally, though, the term “energy harvesting” refers to systems that deliver power for devices operating in the microwatt to low-milliwatt range.
Why it’s Worthwhile
Design engineers who have already embraced the idea have done so for a few reasons. One is to facilitate maintenance-free operation of systems that would otherwise be powered by batteries, which need to be replaced on a regular basis. The approach is especially appealing for systems involving components in remote, hazardous or difficult-to-reach places—as well as for those involving many battery-operated devices.
“Once a lot of sensors are applied, changing the battery is not economical anymore,” says Ruud Vullers of the Holst Centre, which works with clients to create wireless sensor networks incorporating vibration, thermal, photovoltaic (PV) and RF harvesting schemes.
![]() Figure 1. Designers at Leviton Manufacturing produced this wireless occupancy sensor to run on photovoltaics (PV), after measuring light energy in office buildings. They found that 60 to 100 lux is typically available away from windows, and designed their product to run on 40 lux. It can store enough energy to operate up to 48 hours in darkness when fully charged. |
In situations where power would otherwise be provided by a standard electrical system, energy harvesting technology enables reduction of energy and cabling costs, and provides flexibility. For instance, explains Wolfgang Heller, product line manager for EnOcean, the wireless technology allows you to erect or take down walls—to expand or reduce room sizes—without additional cost.
While the initial outlay for energy harvesting is often greater—as much as 1,000% than for battery-based systems, says Abate—that investment pays off over time in multiple ways, because while batteries are relatively inexpensive from a price perspective, “the real value is in the overall cost factor.”
“What many people do not take into account when it comes to batteries is the additional costs and issues beyond the initial price,” he adds, referring to costs involved in replacing and changing, disposal, skilled maintenance labor availability, safety, stocking and inventory maintenance. Abate notes that once these factors are considered for the life of the system, the equation favors the “fit-and-forget, perpetual harvesting solution.” The scale of the project is another factor, because the components are less expensive in quantity. And for companies concerned with earth-friendly operation, Abates says, hazardous material content is an important consideration; energy harvesting is definitely “green.”
Capacity
Vendors of energy harvesting products take various approaches to increasing reliability in case of power source loss. A video by Powercast demonstrates how RF energy emitted by an iPhone can power a sensor, but when no iPhones or other RF energy sources are within range, a dedicated transmitter can step in.
Powercast offers two versions of its original transmitter (1W and 3W), but according to Ostaffe, the company is planning another transmitter designed to conveniently plug into the wall like a nightlight and generate .5W.
In addition, vendors supply storage devices such as capacitors to cache power. This approach enables Seiko’s watch, for example, to accumulate approximately one day of power reserve per 12 hours of wearing time, for up to three months of operation, in its Electricity Storage Unit (ESU). Similarly, Wright and Maddox explain, Leviton designed its WSC04/WSC15 wireless occupancy sensors, which require a minimum of 40 lux to operate, to store enough energy to operate up to 48 hours in darkness when fully charged.
![]() Figure 2. Motion, radiance and heat are just some of the sources of energy tapped by power harvesting technology. (Image courtesy EnOcean) |
Design Considerations
How can a designer determine whether his or her application is appropriate for energy harvesting? In this nascent area, direct communication with suppliers can provide critical information. Most companies billing themselves as power harvesting companies supply to original equipment manufacturers and work closely with customers to achieve their goals.
One thing to keep in mind is that while technologies can often apply across a broad range of applications, young companies target particular markets. According to Heller, EnOcean chose to launch with a focus on building automation because the design cycle is shorter than, say, automotive. That’s why EnOcean’s website is dominated by examples of building automation—even though its products are suited to other applications as well.
![]() Figure 3. An energy harvesting-based wireless sensor network involves multiple sensor modules, each receiving both data and power input, and at least one system radio module. A key factor in powering wireless sensor networks through power harvesting is energy management, as indicated in this diagram, courtesy of EnOcean. |
A good place to start, then, is by considering what sources of power exist in your operating area. From there, develop an understanding of how technologies that tap those sources operate.
“The next step is to determine the power requirements of what you want the energy harvester to power,” says Abate.
Indeed, Wright and Maddox recommend that designers considering energy harvesting “consider power consumption very carefully.” Of course, power consumption depends on the device itself, and the frequency with which it needs to operate—that is, the duty cycle.
“You must then rely on the product data/performance information to determine whether the harvester is even capable of providing the power that will be required,” Abate says, noting it’s also important to “ensure that the time between duty cycles is sufficient enough to allow the harvester to replenish the energy storage mechanism.”
In addition, Abate says, you will need to consider your energy storage methodology.
Finally, “one needs to determine the area or volume that the harvester needs to occupy,” says Vullers. Where space is a key consideration, he notes, the Holst Centre fabricates miniature, microsystems technology-based devices.
To help design engineers understand their needs and plan their designs, Powercast provides a wireless power calculator on its website. In addition, Powercast’s evaluation kits allow designers to experiment with RF energy technology, and development kits facilitate integration of power harvesting into designs. Ostaffe notes that creative antenna design is a key factor in making an installation work. A document on the Perpetuum website, “Getting Started with Vibration Energy Harvesting,” provides useful tips.
![]() Figure 4. A Perpetuum Free Standing Harvester (FSH) powers an industrial wireless sensor node and vibration transducers for the purpose of industrial machine asset monitoring and plant maintenance efficiency. |
It’s in your future
Vullers says that he and his colleagues at the Holst Centre “see more and more ultra low-power devices, which will increase possible energy-harvested applications.”
Likewise, Abate sees a chain of events leading to an eventual explosion in the adoption of energy harvesting: The continued evolution of power-efficient embedded systems and low-power electronics and sensor technologies will facilitate further development of energy harvesting.
As energy harvesting devices continue to proliferate and volumes increase, manufacturing processes will improve. Supply chain and bills of material efficiencies will drive down the investment needed, “and therefore make an even more compelling cost justification for these technologies,” Abate predicts.
Wright and Maddox, too, see the cost issue as important. They anticipate energy harvesting and wireless technology to expand, especially when the cost of these solutions come down. In the meantime, they say the protocol needs to open to allow universal commands for increased flexibility of product types.
EnOcean is pursuing its own version of this vision through the EnOcean Alliance, which Heller says exists to “standardize and internationalize EnOcean wireless technology,” and enable “interoperability between the products of OEM partners.”
Many vendors are continuing their technology and market development plans. Perpetuum, says Abate, is working to ease the processes of evaluating, installing and using the technology—for both end users and OEM partners—and will eventually target applications beyond industrial condition monitoring and rail transportation.
Implementation: A Case Study The Holst Centre’s Ruud Vullers puts special emphasis on power management, noting that “simply replacing batteries by energy harvesting devices will not work. Extra effort is needed to reduce the power consumption of a device. This is a fact that is often not realized by people who consider the use of energy harvesting.” Wright and Maddox corroborate Vullers’ assertion. Although EnOcean’s PTM200C radio transmitter module “is self-contained and made the wall switch transmitter device a purely mechanical design,” they say, the design of their system was a challenge. “Creating a low-power, battery-operated device is one thing; creating an ultra low-power, self-powered wireless device is another.” Because of market requirements, the designers had only about a 2-sq.-in. surface area in rooms as low as 40 lux to collect the power. They decided on photovoltaics (PV) because typically, a significant amount of light goes to waste in offices: “We measured various ceilings and found somewhere in the neighborhood of 60 to 100 lux of lighting—away from windows—which was sufficient for us to utilize solar panels to power our occupancy sensor indefinitely.” Using EnOcean’s PTM240 module helped facilitate the work. “Without these integrated wireless modules utilizing minimal current, this design would not have been possible,” they say. But, they were able to reduce the average current used by the occupancy sensor unit to under to 10uA. Working together with EnOcean engineers, they were able to design a product using the lowest power components possible—around 3 to 5uA continuously. “This was far better than we could accomplish with any off-the-shelf micro,” say Wright and Maddox. Another challenge, they report, was in designing the receiver/transmitter to operate at the greatest distances and overcome outside interference. “Working at 315MHz has been helpful, as there is not much activity/interference in this spectrum,” the pair notes. Still, though, they faced interference challenges at various installation sites. Overcoming them has sometimes been trying, they say, but there are always options available, including adjusting the antenna, or even moving the devices (occupancy sensor, unpowered switch). “There are some situations where wireless won’t work very well—areas with lots of metal, metal enclosure, long distances, etc.,” the conclude. “However, what we have learned is that if you have enough time and patience, this technology can be made to work by making adjustments, moving pieces, finding noise sources, and adding repeaters.” |
More Info:
EnOcean
Holst Centre
Leviton Manufacturing
Perpetuum
Powercast
Seiko
Barbara G. Goode served as editor-in-chief for Sensors magazine for nine years, and currently holds the same position at BioOpticsWorld, which covers optics and photonics for life science applications. Contact her via [email protected].
Subscribe to our FREE magazine,
FREE email newsletters or both!Latest News
About the Author
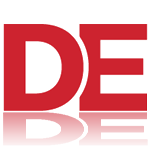
DE’s editors contribute news and new product announcements to Digital Engineering.
Press releases may be sent to them via [email protected].

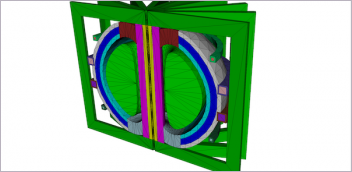
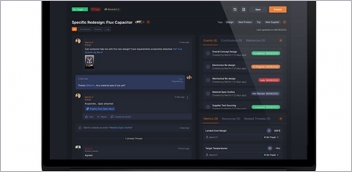
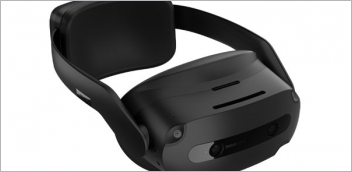